Our group is interested in the general mechanisms by which neuronal oscillations contribute to the organization of information flow and the gating of synaptic plasticity in the human brain. We study the role of specific oscillations for a variety of cognitive functions, such as attention and memory, and during different brain states, such as wakefulness and the various stages of sleep.
To do so, we not only combine multimodal non-invasive neuroimaging and electrophysiological techniques to characterize the oscillatory networks associated with certain neuronal processes and cognitive functions. We also use non-invasive brain stimulation techniques to directly interact with these oscillations to investigate their neurophysiological underpinnings and to manipulate them experimentally in order to unravel their causal contribution.
An important methodological aim of our research is to improve the combination of brain stimulation and neuroimaging both consecutively (offline) and concurrently (online), such as simultaneous TMS-EEG, TUS-EEG, TMS-fMRI, and TUS-fMRI. For example, we study factors confounding the assessment of TMS-evoked EEG potentials (TEPs) and use functional/structural connectivity-informed concurrent TMS-fMRI for the transsynaptic stimulation of deeper brain structures via connected superficial cortical entry sites and proof of target engagement. Another goal is to develop and refine novel interventional approaches including brain state-dependent brain stimulation, such as EEG-triggered TMS to target specific oscillatory brain states (e.g., amplitude levels and phase angles of ongoing neuronal oscillations). We also develop and distribute open-source software and hardware solutions to automate multimodal neurostimulation/-imaging experiments (www.best-toolbox.org) in order to facilitate objectivity, transparency, and reproducibility in the field. Most recently, we also started to explore the potential of robot-navigated transcranial ultrasound stimulation (TUS) for the non-invasive neuromodulation of both cortical and subcortical targets in both healthy human volunteers and psychiatric patient populations.
Research Funding
EIC Pathfinder Project CITRUS (Closed-loop Individualized image-guided Transcranial Ultrasonic Stimulation)
We are joining forces across Europe to advance a new non-invasive technology – transcranial ultrasound stimulation (TUS) – to reversibly modulate brain regions with exquisite millimetre precision, even deep in the brain. As such, we aim to establish an urgently needed novel treatment option for neurological and psychiatric diseases. TUS combines the precision and reach of invasive deep brain stimulation, required to directly target clinically relevant structures, with the non-invasive and low-cost nature of transcranial electromagnetic techniques that are inherently limited in focus and depth. The main roadblock to widespread adoption of TUS in neuroscientific and clinical applications is the difficulty of steering the small ultrasound focus onto the intended target and reaching the desired intensity, with no empirical validation of targeting success currently available. We will develop a neuronavigated TUS-MRI system with advanced magnetic resonance imaging (MRI)-guided application planning and closed-loop application control to enable safe, individualised, and effective high-precision TUS in humans. As such we will unlock the full potential of TUS to non-invasively modulate deep brain structures with unprecedented spatial precision in the millimetre range. The final prototype will be a fully functional device that integrates novel MR-compatible 256-element TUS-transducers (for advanced 3D-steering of the TUS focus) with a custom-tailored 32-channel MR-receiver coil (for accelerated imaging with maximal sensitivity) and closed-loop target validation using MR-acoustic radiation force imaging (MR-ARFI). This novel device with its unique features will enable for the first time the personalized non-invasive high-precision stimulation of cortical and subcortical targets in the human brain. It will be a game changer for both neuroscientific research and clinical application in neurological and psychiatric diseases with the potential to benefit millions of patients.
ACCESS (Advancing central nervous system delivery of therapeutics through focused transcranial ultrasound stimulation-induced opening of the blood brain barrier: establishing a new biotechnology for prevention and therapy of age-associated pathologies)
Functional disorders of the brain, especially age-associated pathological processes, as they also occur in the context of dementia, are still difficult to treat and are associated with enormous personal, social and economic consequences. Conventional pharmacological treatment approaches often reach their limits as the required active ingredients do not cross the blood-brain barrier (BBB) and therefore cannot enter the brain from the blood in the required concentration. New biotechnologies that enable targeted drug delivery to the affected areas of the brain could therefore be a decisive step towards the development of new, innovative therapeutic approaches. The new technology of transcranial ultrasonic stimulation (TUS) allows for the first time the non-invasive, temporary, and localized opening of the BBB (BBBO) in humans, enabling targeted drug delivery. The aim of this project is to establish TUS for BBBO in a translational approach.
Leibniz ScienceCampus “NanoBrain" (Targeted delivery of therapeutics to the brain: advancing translational nanomedicine to decode, prevent, and fight mental disorders)
Stress-related depressive disorders are one of the leading causes of disability and socioeconomic burden. Recent advances in key methodological areas have the potential to revolutionize the understanding of their mechanisms, prevention, and treatment in the near future. First, progress has been made in decoding disease mechanisms, providing evidence for causal involvement of brain derived neurotrophic factor (BDNF) signaling and neuroimmune-mediated disease processes; second, the world-known recent breakthroughs in mRNA technology have spearheaded the development of personalised therapies; and third, advances in nanotechnology have opened up innovative ways for drug delivery to the brain. To break the ground for the development of targeted treatments and preventive strategies for mental disorders, the Leibniz ScienceCampus NanoBrain will combine the translational research expertise at the LIR with major strongholds in the Mainz Life Science community, in particular in the neurosciences and immunotherapy (JGU/UM), nanotechnology (MPI-P), molecular biology (IMB) and RNA-based technology (TRON/BioNTech). During the first funding period, four interdisciplinary core project areas, established between the LIR and collaborating partners will focus on 1) Decoding and targeting stress-associated inflammatory mechanisms, 2) Developing a toolbox for targeted delivery of mRNA-based therapies into the brain using the prototypical stress-associated target BDNF as an example 3) Establishing focused ultrasound stimulation for targeted opening of the blood-brain-barrier and 4) Creating a unique single cell sequencing Resilience Research Resource for the identification of future targets for mRNA-based therapies. NanoBrain will provide an international, vibrant research environment and promote the next generation of young scientists. Ultimately, NanoBrain will be a crucial pillar within the overarching strategy to develop Mainz into a leading centre of excellence in biotechnology.
DFG Research Unit 5434 IADS (Information Abstraction During Sleep ) – Project P08: Transcranial brain stimulation to study memory processing and synaptic plasticity during sleep
This project investigates the oscillatory mechanisms mediating information abstraction and underlying synaptic rescaling during sleep using real-time EEG-triggered transcranial magnetic stimulation (TMS) of the primary motor cortex and transcranial ultrasonic stimulation (TUS) of the thalamus in humans (i) to characterize the excitation-inhibition-balance during sleep spindles and slow oscillations, (ii) to establish spindles/slow oscillations as windows of plasticity that enable synaptic rescaling during sleep, and (iii) to demonstrate that spindles are causally relevant for information abstraction during systems consolidation.
Personalized connectivity- and electric field-guided transcranial modulation of the subgenual anterior cingulate cortex in major depression
The aim of the project is the further development and personalized optimization of repetitive transcranial magnetic stimulation (rTMS) of the left dorsolateral prefrontal cortex (DLPFC) for the treatment of major depression. To this end, we have firstly developed a new optimization method for the positioning/orientation of the TMS coil based on individual mapping of the connectivity between the DLPFC and the subgenual anterior cingulate cortex (sgACC) as well as individual modelling of the TMS-induced electric field in the DLPFC, and secondly, established and optimized an innovative method for individualization of the stimulation intensity which compares the TMS-induced electric fields in motor cortex and DLPFC with reference to the individual motor threshold. A pilot randomized clinical trial in patients with major depression will investigate the feasibility, tolerability and efficacy of our individualized connectivity- and electric field-guided rTMS of the left DLPFC and compare it with state-of-the-art rTMS treatments for major depression. In addition, using simultaneous TMS-fMRI measurements we will also investigate the role of activation and modulation of the sgACC as a mechanism of action of rTMS therapy. In further experiments in healthy volunteers, we will systematically investigate TMS-induced neuronal activation and modulation of the sgACC as a function of DLPFC-sgACC connectivity and stimulation intensity using connectivity- and electric field-guided (r)TMS and simultaneous TMS-fMRI measurements to determine optimal stimulation parameters for the most effective activation and modulation of the sgACC.
Making extinction last: mechanistically understanding and causally probing the role of the ventromedial prefrontal cortex in extinction memory consolidation
Fear extinction consists in learning that a previously threatening stimulus is now safe. Fear extinction establishes a fear-inhibitory safety memory (‘extinction memory’) that needs to be retrieved and expressed when encountering the once-threatening stimulus in order to prevent the recovery of the extinguished fear response. Fear extinction is supposed to underlie the immediate fear/anxiety-reducing effects of exposure-based therapy, and the consolidation of the extinction memories generated during exposure into strong long-term memories is supposed to underlie its long-term beneficial effects. This makes the investigation of the neurophysiological mechanisms underlying extinction memory consolidation a key topic for both the basic neuroscience of learning and memory and for clinical research into fear/anxiety disorders. Based on own previous work, we here focus on the contribution of spontaneous reactivations of neural activity related to extinction learning in the ventromedial prefrontal cortex (vmPFC) during the post-extinction consolidation phase. We aim to determine the electrophysiological correlates of these reactivations, observed in functional magnetic resonance imaging (fMRI), using electroencephalography (EEG) in humans and optical as well as electrophysiological recordings in rats. On this basis, we manipulate reactivations using anatomically precisely targeted tonic and activity-triggered transcranial magnetic stimulation (TMS) in humans and activity-triggered optogenetics in rats, to thus causally probe their effects on extinction consolidation. Experiments will benefit from latest methodological developments in our laboratories and a strictly comparative-translational approach. The project will yield entirely new insights into the mechanisms of extinction memory consolidation and open up new avenues for the neurobiological augmentation of this key resilience and therapy process.
Boehringer Ingelheim Foundation – Establishing methodological excellence in the development of non-invasive neurostimulation procedures.
Many brain structures critical for the treatment of psychiatric disorders are very small and hidden deep in the brain, pushing existing neurostimulation methods (e.g., TMS) to their limits. The innovative method of transcranial ultrasonic stimulation (TUS) now makes it possible for the first time to reach these deep brain structures directly and non-invasively with unprecedented precision and to influence their activity by means of neuromodulation and targeted drug delivery through the blood-brain barrier. Under the direction of the professorship for “Neurostimulation", a fully equipped TUS research laboratory including a robot-guided TUS setup is therefore to be established.
The sensorimotor mu-rhythm as cholinergically controlled pulsed inhibition
The most pronounced neuronal oscillation observable in the electroencephalography (EEG) of the awake and healthy human brain is the 8-14 Hz alpha oscillation. In the sensorimotor cortex, serving as the model region in this project, it is called the mu-rhythm. Initially thought to simply reflect cortical idling, alpha/mu oscillations are nowadays believed to actively gate information flow in the brain. The influential Pulsed Inhibition Hypothesis assumes that the alpha/mu oscillation is asymmetric and composed of recurring bouts of inhibition, which become stronger with increasing amplitude, thereby rhythmically suppressing neural processing in task-irrelevant cortical regions. However, the proposed asymmetric and inhibitory nature of the oscillation has never been directly demonstrated. Moreover, alpha/mu oscillations must be under top-down control of attention, since the mere anticipation of an upcoming stimulus already modulates their amplitude. Yet, also the neuronal implementation of its top-down control is still unknown. It has been hypothesized that cortico-cortical projections from the prefrontal cortex modulate the local release of acetylcholine from ascending basal forebrain cholinergic neurons in the sensory cortices via axo-axonal synapses. Acetylcholine is known to regulate the de /synchronization of neuronal activity. Therefore, prefrontally mediated transient changes in the cholinergic modulation of local sensory alpha/mu oscillations and thus cortical excitability may represent the neural mechanism, underlying the attentional gating of perception as well as resulting stimulus-induced synaptic plasticity. I will test the hypotheses of pulsed inhibition and a prefrontally controlled cholinergic alpha modulation for the model case of the sensorimotor mu-rhythm. The combination of transcranial magnetic stimulation (TMS) of the primary motor cortex with concurrent EEG assessment of the sensorimotor mu-rhythm allows to noninvasively study cortical excitability and intra-cortical inhibition in an amplitude and phase-dependent manner in the human brain. The project is composed of two major parts. Firstly, I will use real-time EEG-triggered single- and paired-pulse TMS to study the neural mechanisms mediating fluctuations in phase and amplitude of the spontaneous sensorimotor mu-rhythm and their implication for the induction of synaptic plasticity. Secondly, I will employ (anti )cholinergic pharmacological interventions and a virtual lesion approach using repetitive TMS to uncover the neural mechanisms mediating top-down control of the mu-rhythm in the context of a tactile spatial attention task. Together the project is expected to provide fundamentally new insights into the neurophysiological underpinnings of the alpha/mu oscillations, their role in gating information processing and synaptic plasticity, and the prefrontal and cholinergic mechanisms of their attentional top-down control.
Our core research interest is in the function of neuronal oscillations in the waking and the sleeping human brain.
Funding Partners
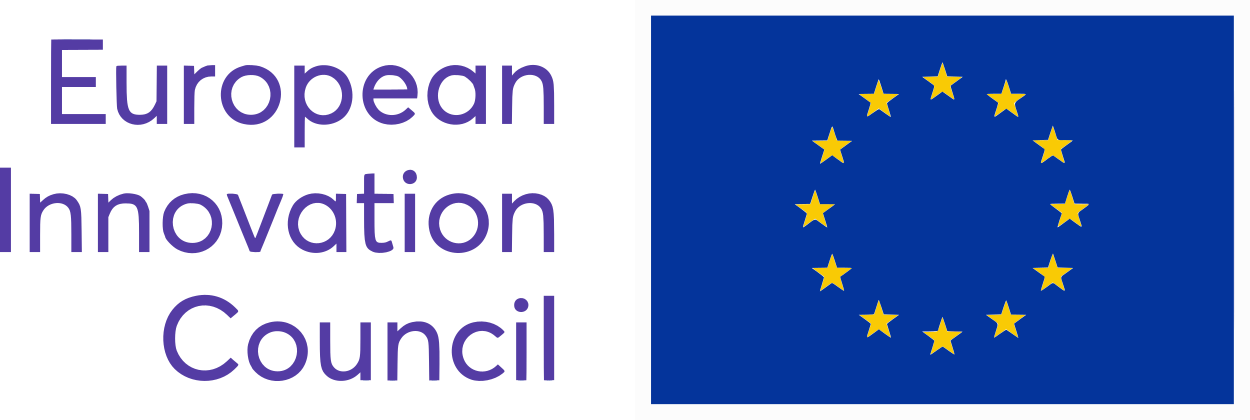

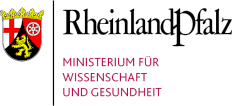

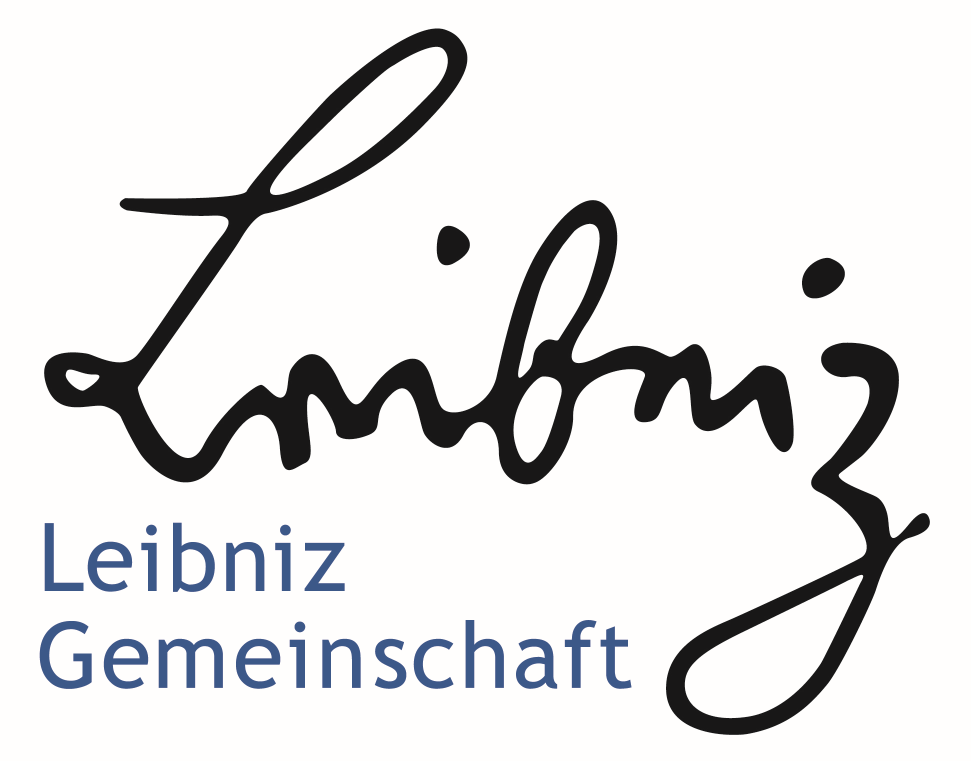